Salk researchers uncover new function of mitochondria
Mitochondria, tiny structures present in most cells, are known for their energy-generating machinery. Now, Salk researchers have discovered a new function of mitochondria: they set off molecular alarms when cells are exposed to stress or chemicals that can damage DNA, such as chemotherapy. The results, published online in Nature Metabolism on December 9, 2019, could lead to new cancer treatments that prevent tumors from becoming resistant to chemotherapy.
Most of the DNA that a cell needs to function is found inside the cell's nucleus, packaged in chromosomes and inherited from both parents. But mitochondria each contain their own small circles of DNA (called mitochondrial DNA or mtDNA), passed only from a mother to her offspring. And most cells contain hundreds--or even thousands--of mitochondria.
Shadel's lab group previously showed that cells respond to improperly packaged mtDNA similarly to how they would react to an invading virus--by releasing it from mitochondria and launching an immune response that beefs up the cell's defenses.
In the new study, Shadel and his colleagues set out to look in more detail at what molecular pathways are activated by the release of damaged mtDNA into the cell's interior. They homed in on a subset of genes known as interferon-stimulated genes, or ISGs, that are typically activated by the presence of viruses. But in this case, the team realized, the genes were a particular subset of ISGs turned on by viruses. And this same subset of ISGs is often found to be activated in cancer cells that have developed resistance to chemotherapy with DNA-damaging agents like doxyrubicin.
To destroy cancer, doxyrubicin targets the nuclear DNA. But the new study found that the drug also causes the damage and release of mtDNA, which in turn activates ISGs. This subset of ISGs, the group discovered, helps protect nuclear DNA from damage--and, thus, causes increased resistance to the chemotherapy drug. When Shadel and his colleagues induced mitochondrial stress in melanoma cancer cells, the cells became more resistant to doxyrubicin when grown in culture dishes and even in mice, as higher levels of the ISGs were protecting the cell's DNA.
"Perhaps the fact that mitochondrial DNA is present in so many copies in each cell, and has fewer of its own DNA repair pathways, makes it a very effective sensor of DNA stress," says Shadel.
Most of the time, he points out, it's probably a good thing that the mtDNA is more prone to damage--it acts like a canary in a coal mine to protect healthy cells. But in cancer cells, it means that doxyrubicin--by damaging mtDNA first and setting off molecular alarm bells--can be less effective at damaging the nuclear DNA of cancer cells.
"It says to me that if you can prevent damage to mitochondrial DNA or its release during cancer treatment, you might prevent this form of chemotherapy resistance," Shadel says.
His group is planning future studies on exactly how mtDNA is damaged and released and which DNA repair pathways are activated by the ISGs in the cell's nucleus to ward off damage.
Wu, Z., et al. (2019) Mitochondrial DNA stress signalling protects the nuclear genome. Nature Metabolism. doi.org/10.1038/s42255-019-0150-8.
Mitochondria are the 'canary in the coal mine' for cellular stress
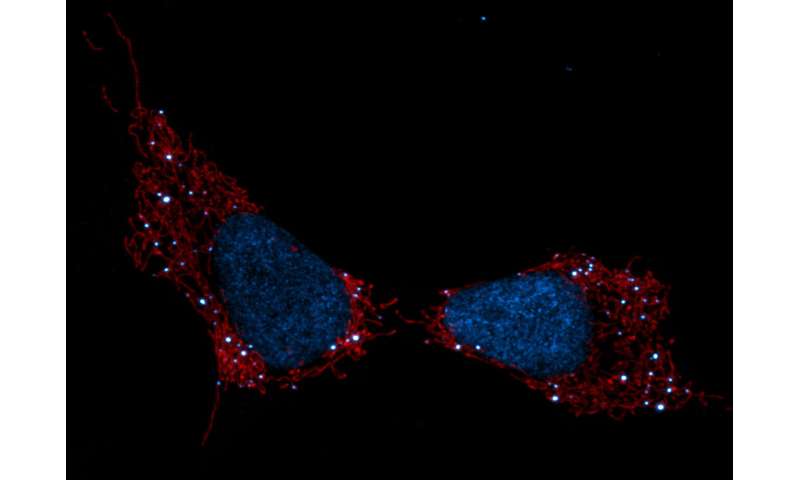
Mitochondria, tiny structures present in most cells, are known for their energy-generating machinery. Now, Salk researchers have discovered a new function of mitochondria: they set off molecular alarms when cells are exposed to stress or chemicals that can damage DNA, such as chemotherapy. The results, published online in Nature Metabolism on December 9, 2019, could lead to new cancer treatments that prevent tumors from becoming resistant to chemotherapy.
"Mitochondria are acting as a first line of defense in sensing DNA stress. The mitochondria tell the rest of the cell, 'Hey, I'm under attack, you better protect yourself,'" says Gerald Shadel, a professor in Salk's Molecular and Cell Biology Laboratory and the Audrey Geisel Chair in Biomedical Science.
Most of the DNA that a cell needs to function is found inside the cell's nucleus, packaged in chromosomes and inherited from both parents. But mitochondria each contain their own small circles of DNA (called mitochondrial DNA or mtDNA), passed only from a mother to her offspring. And most cells contain hundreds—or even thousands—of mitochondria.
Shadel's lab group previously showed that cells respond to improperly packaged mtDNA similarly to how they would react to an invading virus—by releasing it from mitochondria and launching an immune response that beefs up the cell's defenses.
In the new study, Shadel and his colleagues set out to look in more detail at what molecular pathways are activated by the release of damaged mtDNA into the cell's interior. They homed in on a subset of genes known as interferon-stimulated genes, or ISGs, that are typically activated by the presence of viruses. But in this case, the team realized, the genes were a particular subset of ISGs turned on by viruses. And this same subset of ISGs is often found to be activated in cancer cells that have developed resistance to chemotherapy with DNA-damaging agents like doxyrubicin.
To destroy cancer, doxyrubicin targets the nuclear DNA. But the new study found that the drug also causes the damage and release of mtDNA, which in turn activates ISGs. This subset of ISGs, the group discovered, helps protect nuclear DNA from damage—and, thus, causes increased resistance to the chemotherapy drug. When Shadel and his colleagues induced mitochondrial stress in melanoma cancer cells, the cells became more resistant to doxyrubicin when grown in culture dishes and even in mice, as higher levels of the ISGs were protecting the cell's DNA.
"Perhaps the fact that mitochondrial DNA is present in so many copies in each cell, and has fewer of its own DNA repair pathways, makes it a very effective sensor of DNA stress," says Shadel.
Most of the time, he points out, it's probably a good thing that the mtDNA is more prone to damage—it acts like a canary in a coal mine to protect healthy cells. But in cancer cells, it means that doxyrubicin—by damaging mtDNA first and setting off molecular alarm bells—can be less effective at damaging the nuclear DNA of cancer cells.
"It says to me that if you can prevent damage to mitochondrial DNA or its release during cancer treatment, you might prevent this form of chemotherapy resistance," Shadel says.
His group is planning future studies on exactly how mtDNA is damaged and released and which DNA repair pathways are activated by the ISGs in the cell's nucleus to ward off damage.
News source: https://phys.org
New Fluorescent Marker Allows Detailed Study of Mitochondria in Live Cells, Study Reports
MitoPB Yellow, a new fluorescent specific marker, allows scientists to study live mitochondria, possibly leading to a better understanding of mitochondrial diseases and to new treatments, researchers in Japan report.
The marker is much more stable than current markers, and allows mitochondria to be studied for extended periods without inducing toxicity to living cells.
The study, “A photostable fluorescent marker for the superresolution live imaging of the dynamic structure of the mitochondrial cristae,” was published in the journal PNAS.
Mitochondria, the small cellular structures that produce energy for our cells, are made of an inner and an outer membrane. The enzymes that produce ATP (the molecular “currency” of energy) are located on the inner membrane. This membrane is folded into structures known as cristae, which increase its surface area.
STED microscopy is a type of super-resolution microscopy that allows a detailed look into the interior of cells. For this technique, researchers use fluorescent dyes to see the structures inside cells. But current dyes are not optimal, as they stop fluorescing with time, a process called photobleaching. This prevents researchers from observing cells over extended periods, and damaged markers can cause cells to die.
Researchers at Nagoya University’s Institute of Transformative Bio-Molecules have developed a new marker molecule, called MitoPB Yellow, which has a much longer lifespan under the microscope. This marker is specific for the inner mitochondrial membrane, making the mitochondria visible for longer periods of time.
Furthermore, the new marker allows researchers to detect structural changes of the mitochondrial inner membrane in live cells. Before, these structural changes could only be seen in dead cells.
In this study, researchers compared the MitoPB Yellow to other mitochondrial fluorescent markers. MitoPB Yellow showed greater stability — even after 50 images had been taken using the microscopy, researchers saw no significant decrease in the fluorescence intensity. Moreover, the intensity of MitoPB Yellow was maintained above 70%, the highest rating among the different mitochondrial markers.
To demonstrate the usefulness of MitoPB Yellow for imaging live cells, researchers placed mitochondria under conditions that are known to induce structural changes, which became visible using MitoPB Yellow.
Changes in mitochondria in response to deprivation of nutrients were also observed in great detail (high-resolution).
In this stressful environment, the inner mitochondrial membranes fused together both within a single mitochondrion and between neighboring mitochondria. This process may increase energy production while protecting these cellular structures from degradation.
By allowing for a more detailed study of live mitochondria, MitoPB Yellow may aid in understanding, diagnosing and developing therapies for mitochondrial disease.
“In summary, MitoPB Yellow surpasses currently used fluorescent mitochondrial markers,” the researchers wrote.
News source: https://mitochondrialdiseasenews.com
Know thy mitochondria: Autoimmunity to organelles and their DNA
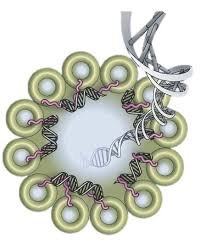
VDAC1 oligomerization forms a pore for mtDNA. Credit: Jeonghan Ki
The immune system uses its mitochondria to self-stimulate innate and adaptive responses to infection. Reactive oxygen species (ROS), immunogenic mitochondrial DNA (mtDNA), and even whole mitochondria are locally mobilized in a delicate balance to create hot spots of inflammatory action. When normal limiting feedback on these processes is compromised, destructive autoimmune reactions often arise.
One common sign that all is not well in the immune system is the presence of antimitochondrial antibodies (AMAs) in the blood. In systemic lupus erthyematosis (SLE), for example, AMAs to several mitochondrial compartments can be found. Some AMAs target proteins normally found in the outer mitochondrial membrane while others seek out mtDNA. The question that naturally arises here is how the immune system sees mtDNA if it normally lives deep inside the mitochondrial matrix.
One answer was provided in a recent paper appearing in Science that shows released mtDNA can lead to lupus. In a nutshell, when mitochondria are stressed in various ways, mtDNA breaks into fragments and then binds to voltage-dependent anion channels (VDACs) in the outer membrane. This causes individual VDAC monomers to aggregate and form a meta-pore in their center through which the mtDNA can escape. Once in the cytoplasm, various nonspecific sensors including Toll receptors for single-stranded DNA, and the GAS-STING pathways for double-stranded DNA unleash a full-blown type I interferon (IFN) response.
Each VDAC monomer itself contains a highly-tuned channel that passes critical molecules of different sizes and charges in either direction according to the current prevailing membrane potential. Eliminating VDAC function entirely is incompatible with higher forms of eukaryotic life. Fortunately, the authors found that lupus-like immune activation can be eliminated by blocking just one form of the channel, the VDAC1 variety, with the oligomerization inhibitor VBIT-4.
The celebrity singer Seal suffers from discoid lupus erythematosis. It is a cutaneous form of lupus that is frequently related to SLE. As a general treatment strategy, interfering with mtDNA release alone might not fully eliminate all the AMAs someone like Seal might possess. However, for other forms of the disease, like lupus nephritis, such an approach might be more useful since all the AMAs found appear to be against double stranded mtDNA.
Other kinds of autoimmune disease, like those affecting the bile ducts of the liver, can similarly involve AMAs. Primary biliary cholangitis and primary sclerosing cholangitis are two such diseases that are characterized by different forms of autoantibodies. Sclerosing cholangitis, endured by the great NFL running back Walter Payton, is associated with antinuclear antibodies. On the other hand, biliary cholangitis sufferers have AMAs against the lipoate containing the E2 subunit of the pyruvate dehydrogenase complex. Additionally, they often also have antibodies to enzymes like sulfite oxidase and glycogen phosphorylase that are commonly associated to liver mitochondria.
At this point in time, it is not known exactly how and where these particular kinds of antibodies are made. The immunogenic E2 subunit normally floats inside the mitochondrial matrix along with the mtDNA and does not habitually escape through any channels. Presumably, errant pieces of degraded mitochondria from expiring cells could be contributing to antibody formation, as could whole mitochondria purposively ejected from cells.
One glaring problem in trying to understand the generation of AMAs that we have overlooked here so far is how the mtDNA gets past the inner membrane to reach the outer membrane VDAC. I asked Peggy Crow, the author of the accompanying opinion piece to the main article, to comment on research in this area. She notes that although the exact answer is not known, imaging studies have revealed another pore system that acts much in parallel to VDAC. These so-called "BAK/BAX macropores' permit the inner mitochondrial membrane to herniate into the cytosol, permeabilize, and ferry matrix components, including mtDNA.
We haven't yet said much about the reactive oxygen species component of mitochondrial inflammation mentioned above. There is another recent finding that ties together oligomerization of the VDAC1 channel and ROS. More specifically, the authors of the study found that a molecule known as lipoxstatin-1 protects cells against ROS damage by reducing VDA1 and restoring levels of the enzyme GPX4.
GPX4 is a unique selenium-utilizing form of glutathione peroxidase that specifically protects cytosolic membrane lipids from oxidative damage. When GPX4 is compromised, a unique form of apoptosis known as ferroptosis occurs throughout the cell. By blocking oligomerization of VDAC1, and not VDAC2 or 3, liproxstatin-1 was found to short circuit the ferroptosis pathway.
Importantly, liproxistatin also blocked the shrinkage of mitochondria, reduction and disruption of their cristae, and rupture of the outer mitochondrial membrane that keynotes ferroptosis. GPX4 deficiency is not an autoimmune disease but rather a disease of runaway ROS damage and ferroptosis within extremely circumscribed cell populations. It is extremely rare—so rare, in fact, that when one patient was recently diagnosed, he was the only person in the world to have this enigmatic disease.
News source: https://medicalxpress.com/
Autism Spectrum Disorders and Mitochondria
Mitochondria are the powerhouse that generate over 90% of the ATP produced in cells. In addition to its role in energy production, the mitochondrion also plays a major role in carbohydrate, fatty acid, amino acid and nucleotide metabolism, programmed cell death (apoptosis), generation of and protection against reactive oxygen species (ROS), immune response, regulation of intracellular calcium ion levels and even maintenance of gut microbiota. With its essential role in bio-energetic as well as non-energetic biological processes, it is not surprising that proper cellular, tissue and organ function is dependent upon proper mitochondrial function. Accordingly, mitochondrial dysfunction has been shown to be directly linked to a variety of medical disorders, particularly neuromuscular disorders and increasing evidence has linked mitochondrial dysfunction to neurodegenerative and neurodevelopmental disorders such as Alzheimer's Disease (AD), Parkinson's Disease (PD), Rett Syndrome (RS) and Autism Spectrum Disorders (ASD). Over the last 40 years there has been a dramatic increase in the diagnosis of ASD and, more recently, an increasing body of evidence indicates that mitochondrial dysfunction plays an important role in ASD development. In this review, the latest evidence linking mitochondrial dysfunction and abnormalities in mitochondrial DNA (mtDNA) to the pathogenesis of autism will be presented. This review will also summarize the results of several recent `approaches used for improving mitochondrial function that may lead to new therapeutic approaches to managing and/or treating ASD.
Abstract link: 10.1016/j.pnpbp.2018.12.015
During the Targeting Mitochondria 2019 Congress, we will talk about the relation between Autism Spectrum Disorders and Mitochondria.
For more info: https://targeting-mitochondria.com/